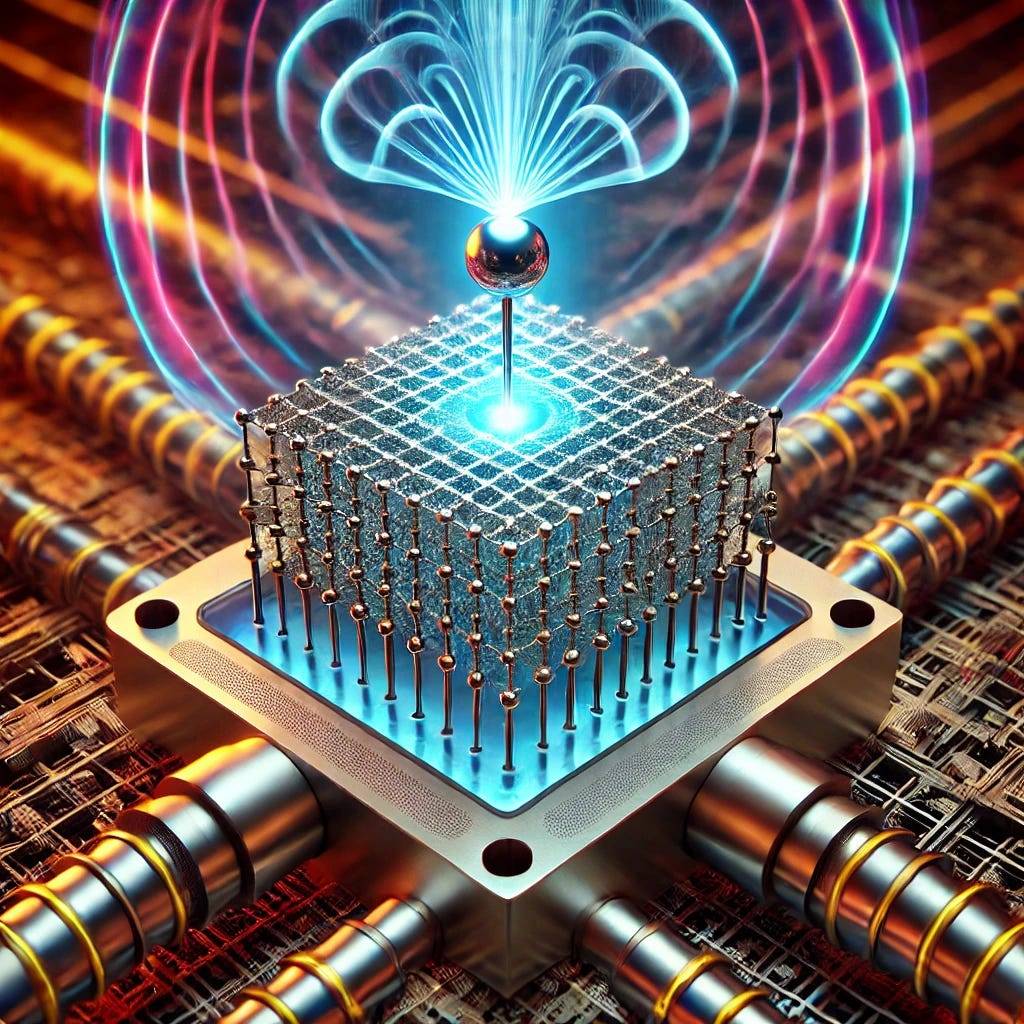
Quantum computing, a field once confined to theoretical musings, is now on the brink of revolutionary breakthroughs. Central to its progress are single-qubit gates, foundational operations essential for error-tolerant quantum computation. Researchers achieved a staggering milestone: single-qubit gates with error rates at the 10^-7 level. This feat, achieved using trapped-ion 43Ca+ hyperfine clock qubits, challenges the boundaries of precision and sets a new benchmark for quantum fidelity. As quantum gates flirt with perfection, they illuminate paths toward fault-tolerant quantum systems and practical large-scale computation.
High-Fidelity Gates: The Key to Quantum Stability
Quantum algorithms rely heavily on the precision of their building blocks: single- and multi-qubit gates. A qubit, the quantum analog of a classical bit, encodes information in states |0⟩ and |1⟩. The accuracy of operations on these states defines the overall reliability of a quantum processor.
Trapped ions, like 43Ca+, have emerged as champions in the quest for fidelity due to their “atomic clock” transitions. These transitions remain stable against magnetic field fluctuations, extending coherence times to over an hour. Such stability is critical in reducing errors caused by decoherence and environmental noise. At an error rate of just 1.5(4) × 10^-7, the experiment described in the document showcases an unprecedented combination of speed and accuracy, setting it apart from other quantum platforms.
Moreover, the use of chip-integrated microwave resonators for qubit manipulation introduces remarkable efficiency. This technology eliminates the reliance on laser-driven controls, offering both scalability and cost-effectiveness.
Why Lowering Gate Errors Transforms Quantum Computing
Error suppression in quantum gates is no trivial feat. The researchers identified and mitigated multiple error sources, including:
- Decoherence: Errors from qubit state loss due to interaction with the environment.
- Leakage and Bit-Flips: Unintended transitions outside the computational subspace.
- Amplitude Noise: Variations in the control signal that distort qubit rotations.
The following graph illustrates the relative contributions of various error sources during a single 13 μs quantum gate, providing a clear view of the dominant and minimized factors.
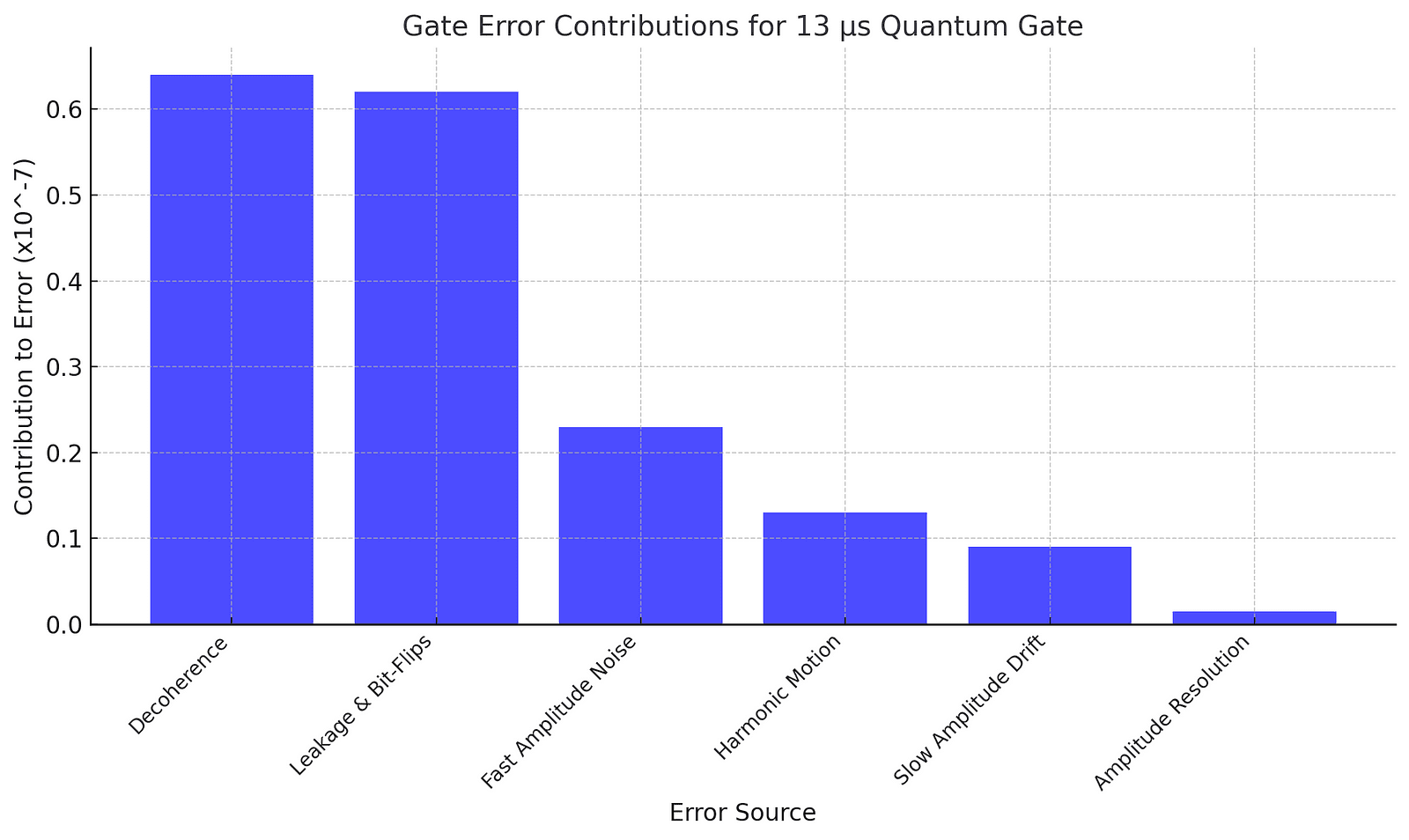
By employing sophisticated calibration methods, including randomized benchmarking, the team quantified and reduced these errors. Interleaving calibrations with gate operations every minute maintained optimal conditions, countering slow amplitude drifts caused by thermal fluctuations in the system.
The Implications for Quantum Error Correction
Achieving error rates below 10^-7 is a game-changer for error correction. Quantum error correction (QEC) protocols require gate fidelities exceeding 99.9% to offset the overhead of maintaining logical qubits. Lower error rates reduce the number of physical qubits needed for each logical qubit, making fault-tolerant systems more feasible.
For instance, decoherence — a leading error source — can be mitigated using dynamical decoupling techniques. These involve frequent, precise qubit rotations to cancel out environmental noise, akin to spinning a gyroscope for stability. With such strategies, even coherence times of 70 seconds can be extended effectively.
Furthermore, the integration of high-fidelity microwave-controlled gates positions trapped ions as leading candidates for scalable quantum processors. Unlike superconducting circuits or neutral atoms, trapped ions offer unmatched coherence and precise control, making them ideal for both academic exploration and commercial applications.
How Machines Learn to Think
Trapped-ion qubits excel not only in computation but also in sensing applications, where their stability enables precise measurements of physical constants.
Decoherence: A Double-Edged Sword
Decoherence is both a challenge and a diagnostic tool. By analyzing decoherence rates, researchers can identify and mitigate systemic weaknesses.
Scaling Down to Scale Up
The chip-integrated microwave resonator used in this experiment demonstrates how miniaturization drives both precision and scalability in quantum hardware.
Breaking the Laser Dependency
Microwave-driven gates reduce reliance on costly and complex laser systems, offering a pathway to more accessible quantum technologies.
The Path Forward
Combining low error rates with fast gate speeds (e.g., 4.4 μs for the fastest gates) provides a blueprint for efficient quantum architectures capable of handling real-world problems.
The Dawn of Fault-Tolerant Systems
The achievement of sub-part-per-million error rates in single-qubit gates is more than a technical triumph — it’s a step toward a practical quantum future. By combining extraordinary precision with scalable design, researchers are paving the way for quantum computers capable of tackling intractable problems in cryptography, optimization, and material science.
As we continue to refine the art of error correction and quantum gate fidelity, the dream of fault-tolerant quantum computing inches closer to reality. These breakthroughs remind us that the limits of today are merely the starting points of tomorrow’s innovations.
About Disruptive Concepts
Welcome to @Disruptive Concepts — your crystal ball into the future of technology. 🚀 Subscribe for new insight videos every Saturday!
See us on https://twitter.com/DisruptConcept
Read us on https://medium.com/@disruptiveconcepts
Enjoy us at https://disruptive-concepts.com
Whitepapers for you at: https://disruptiveconcepts.gumroad.com/l/emjml