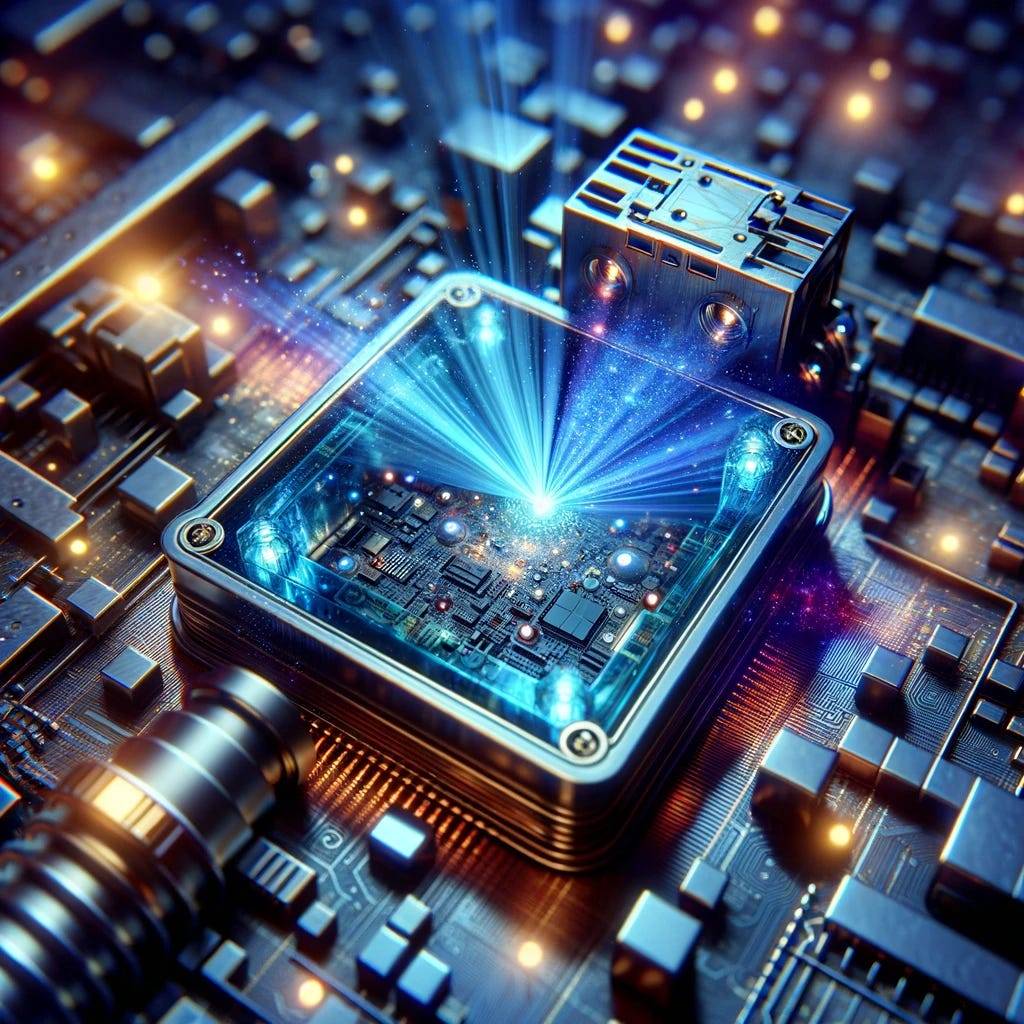
Silicon photomultipliers (SiPMs) stand at the forefront of a technological revolution in photon detection, particularly at cryogenic temperatures. This technology, crucial for experiments in particle physics, astronomy, and medical imaging, allows us to see the invisible — photons that our eyes, and most cameras, cannot detect. By enhancing our ability to capture these elusive particles, SiPMs help unlock mysteries of the universe, from the behavior of dark matter to the intricacies of the human body. Imagine a world where we can visualize the faintest glimmers of light from the farthest reaches of space or detect early signs of diseases with unprecedented clarity — this is the promise of SiPM technology.
The Experimental Journey
Researchers have crafted an experimental setup to measure how SiPMs perform in the chilling embrace of liquid nitrogen. This scenario mimics the extreme conditions these detectors often face in high-tech applications, such as in space telescopes or in the icy grips of Antarctic observatories. By understanding how SiPMs behave at these temperatures, scientists can refine their designs, leading to more sensitive and accurate detectors. This not only advances research but also paves the way for practical applications that could one day result in gadgets that allow us to peer through walls or detect environmental pollutants with a simple handheld device.
The Pulse of Progress
Through meticulous data acquisition and processing, the researchers capture and analyze the faint signals produced by photons striking the SiPMs. Each detected photon can be the difference between an ordinary understanding and a breakthrough. The data tell stories of photons’ journeys across space or through materials, helping scientists map out phenomena with precision. This process is akin to listening to the faint whispers of the universe, where each photon provides clues to the cosmic puzzles scientists are eager to solve.
Unveiling the Invisible
The findings reveal that SiPMs are less efficient at colder temperatures, a challenge that needs addressing to optimize their use in cryogenic environments. This discovery is vital for experiments that rely on ultra-cold conditions, such as those searching for neutrinoless double-beta decay — a process that, if detected, could explain why our universe is made of matter and not antimatter. It’s like finding the ghostly fingerprints of particles that shaped the cosmos, a quest that could redefine our understanding of the universe’s fundamental laws.
To see how SiPMs perform under different conditions, let’s look at a graph below comparing their efficiency at room temperature and when they’re cooled down with liquid nitrogen.
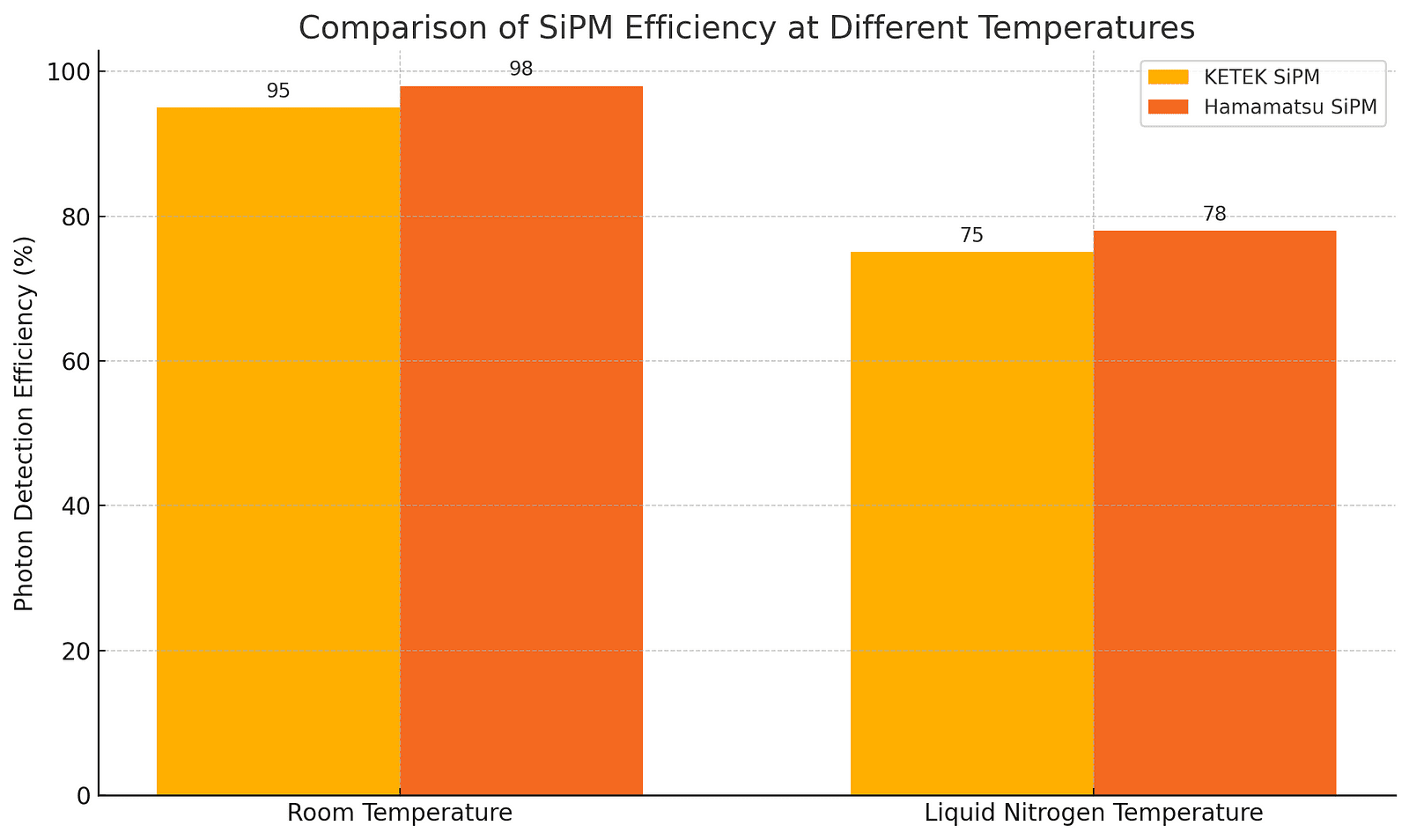
Pioneering the Future
The study not only provides a deeper understanding of SiPM behavior in extreme conditions but also charts a course for future improvements. By identifying the specific limitations of current technologies, researchers can innovate new solutions that enhance photon detection efficiency. This could lead to more sensitive detectors capable of capturing photons from the most elusive sources — be it the faint glow of distant stars or the weak signals indicative of rare physical phenomena.
Single-Photon Sensitivity
SiPMs can detect single photons, distinguishing light levels that would be invisible to traditional detectors. This sensitivity is crucial for applications in quantum computing and deep-space communications, where every photon counts.
Nanosecond Timing
These detectors can time the arrival of photons with nanosecond precision. This capability is essential for high-resolution imaging techniques used in medical diagnostics, such as PET scans, enabling doctors to see how diseases affect the body in real time.
Resistance to Magnetic Fields
Unlike many other light detectors, SiPMs operate flawlessly in strong magnetic fields. This makes them indispensable in particle physics experiments, where strong magnetic fields are used to steer particles.
Versatility Across the Spectrum
SiPMs are sensitive to a wide range of light wavelengths, from ultraviolet to near-infrared. This adaptability makes them suitable for a plethora of scientific and commercial applications, including art restoration and forensic investigations.
Compact and Robust
Their solid-state design makes SiPMs compact and robust, unlike the bulky and fragile photomultiplier tubes traditionally used for similar purposes. This durability is critical for deploying detectors in harsh environments, such as on spacecraft or under the sea.
A Beacon of Hope
The journey of silicon photomultipliers from lab benches to the edge of space embodies the relentless human quest for knowledge. These tiny detectors, capable of capturing the faintest whispers of light, hold the key to unraveling some of the universe’s greatest mysteries. For a generation poised on the brink of technological wonders, SiPMs represent not just tools of scientific inquiry but beacons of hope — promising deeper insights into the fabric of reality and empowering us to solve challenges from healthcare to environmental monitoring. As we continue to explore the cosmos and the quantum realms, SiPMs light the way, proving that even the smallest components can have an astronomical impact on our understanding of the universe.
About Disruptive Concepts
https://www.disruptive-concepts.com/
Welcome to @Disruptive Concepts — your crystal ball into the future of technology. 🚀 Subscribe for new insight videos every Saturday!